InGaAs Wafers
The so-called InGaAs material refers to a complete epitaxial stack composed of a variety of layers, with InGaAs forming the key part – the absorption – and being responsible for the material’s optical properties. The InGaAs layer itself is a III-V semiconductor that belongs to the family of In(x)Ga(1-x)As(y)P(1-y). For binary GaAs, InP, GaP, or InAs, the x and y are set to 0 or 1. In quaternary InGaAsP and ternary InGaAs a variety of x and y values are possible, and each combination will tune the semiconductor to different applications.
Adjusting the proportion of Indium to Gallium and Arsenic to Phosphorus affects the energy band-gap and lattice parameter of the compound. By the rule, as given by Planck equation E=hν, the higher the energy the higher the frequency, which is inversely proportional to the wavelength. An adjustment of x in In(x)Ga(1-x)As allows to lower the energy band gap and therefore make the light spectral range longer, but this happens at the cost of changing the lattice constant and mismatching to an InP substrate. The lattice constant is a key value to designing the epitaxial stack and predicting the strain that can occur in the layers.
Cutt-off Wavelength
The quaternary InGaAsP, which would be the result of mixing all four chemical elements, finds its usage in the 1.0-1.6um range, as relevant band-gaps will be achieved when lattice-matching InGaAsP to InP. Omitting the Phosphorus (y=1), to create the ternary InGaAs, would allow to lattice-match to InP at the composition of Indium at 53% – In(0.53)Ga(0.47)As – with energy band gap of 0.73eV equal to cut-off wavelength of around 1.7um.
Cut-off wavelength means that only wavelengths shorter than the given value will be absorbed and any values above will have very low or none detectivity; a parameter measured as D* (cm Hz^1/2W^-1) and describing a normalized signal-to-noise ratio of a device.
Standard InGaAs vs. Extended InGaAs
InGaAs materials with InGaAs in the absorption layer and cut-off wavelength set at 1.7um (lattice-matched to InP) are often referred to as Standard, while materials with cut-off above 1.7um (lattice-mismatched) are called Extended.
The reason to extend the detection range to higher values, such as to 2.6um, is simple and answers the need of an application, but the execution imposes a great challenge. A lattice-mismatch requires a specially designed and graded buffer layer, which will act as a bridge between the substrate and the absorber. This solution is, however, never ideal, and a number of misfit defects and anomalies in dark current can occur. As such, once developed, the buffer layer is often considered the company’s secret.
Epitaxial stack design
When developing the epitaxial stack, different InP substrates (N-type or Semi-Insulating) can be used depending on the contacting design. A buffer & contact layer is grown on the substrate and then followed by the InGaAs absorption layer with a doping level and thickness optimized to the operating wavelength. Depending on the desired device properties as well as cutoff wavelength choice, the epitaxial stack can consist of additional layers such as space-charge region, interfaces, and a cap, which are responsible for controlling carrier recombination, drift, and diffusion as well as preventing surface current leakage from occurring. The final step is applying a P-type contact at the top of the epitaxial stack. Such contact is commonly created by a Zn diffusion process, which forms a P-region inside the intrinsic InGaAs layer.
Material performance
In a detector, a reverse voltage bias is applied, which means that no current is intended to flow if the device is not illuminated. However, a small current, called dark current, will always be present due to random recombination that happens inside the depletion region. As the dark current is the main source of noise in a detector, the lower the value that can be achieved from a specific material – the better. Another quality of the InGaAs semiconductor is a density of misfit and surface defects. This is particularly important for focal plane arrays (FPAs), where a larger surface area is selected to prepare a matrix of pixels, with as many as possible providing a correct signal on the read-out circuit. Surface issues and misfit defects can negatively influence a wide range of device’s electro-optical properties and thus are generally undesirable.
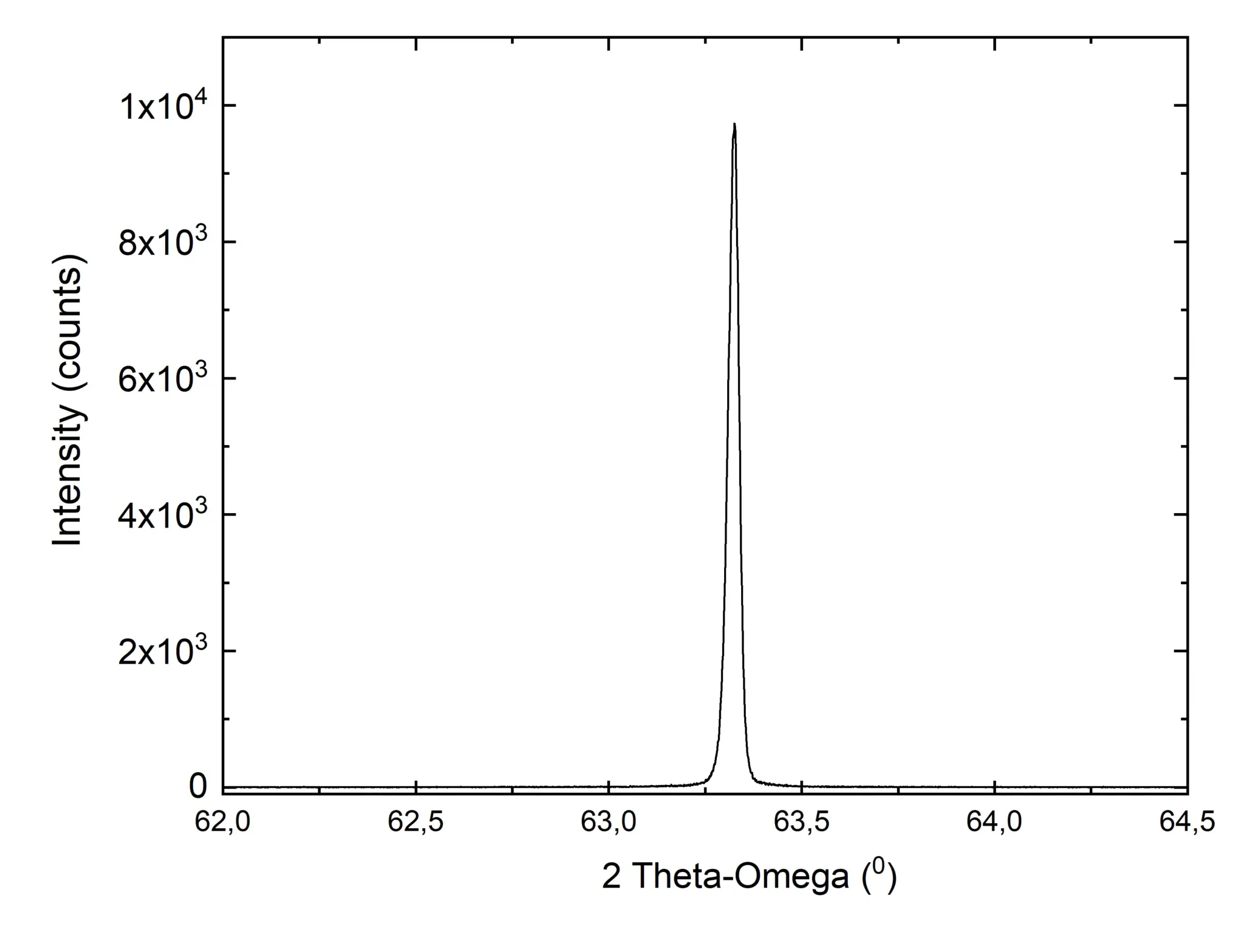
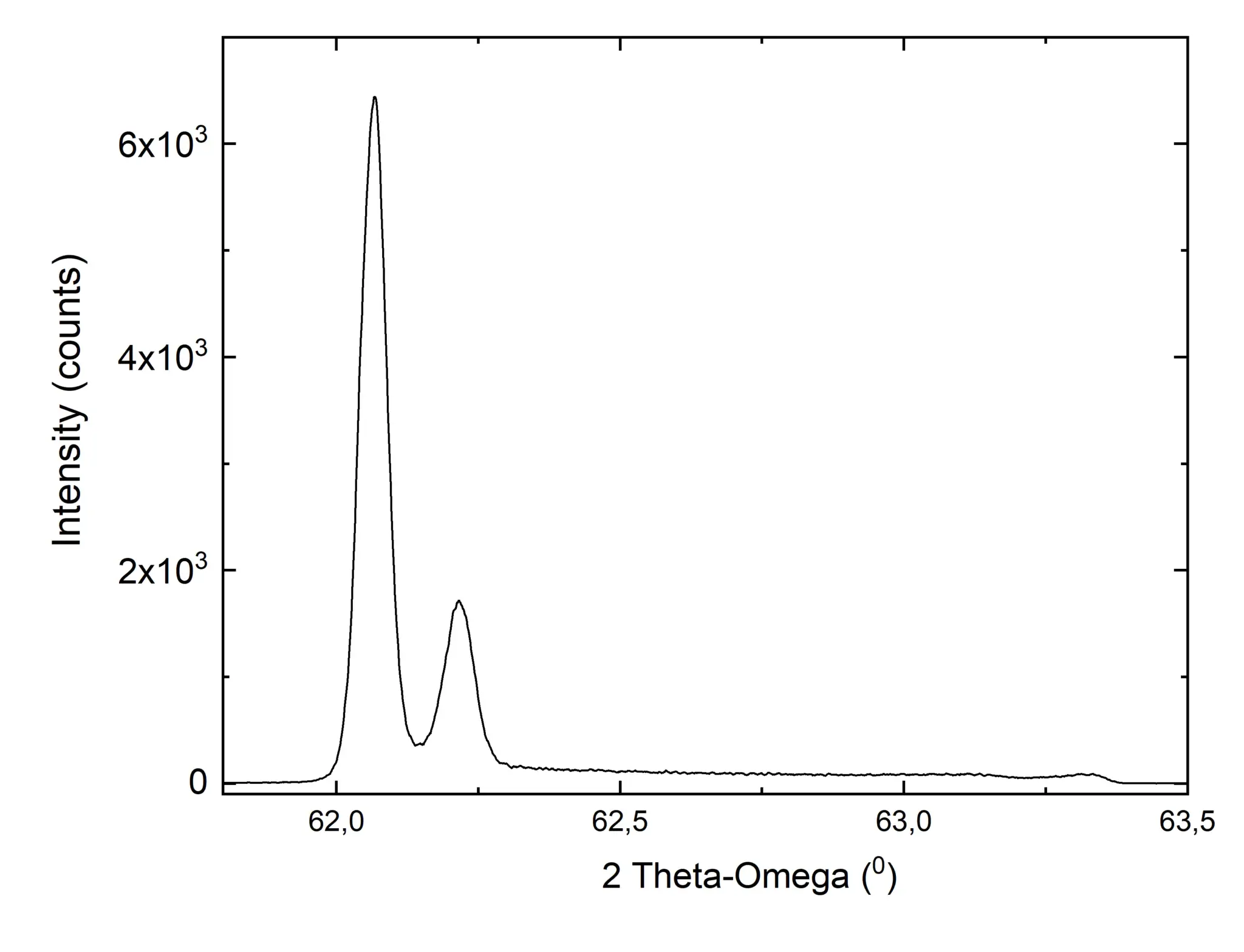
For wavelengths of Standard InGaAs (1.7um), very low doping levels may be desired. Due to a presence of impurities in epitaxy reactors, a truly undoped material is very difficult to achieve. The SIMS measurement below shows a doping level in our InGaAs absorber (green line) below the detection level, so less than 10^15. Similarly, our ECV measurement was able to detect the carrier concentration as low as 1.6E14/cm3.
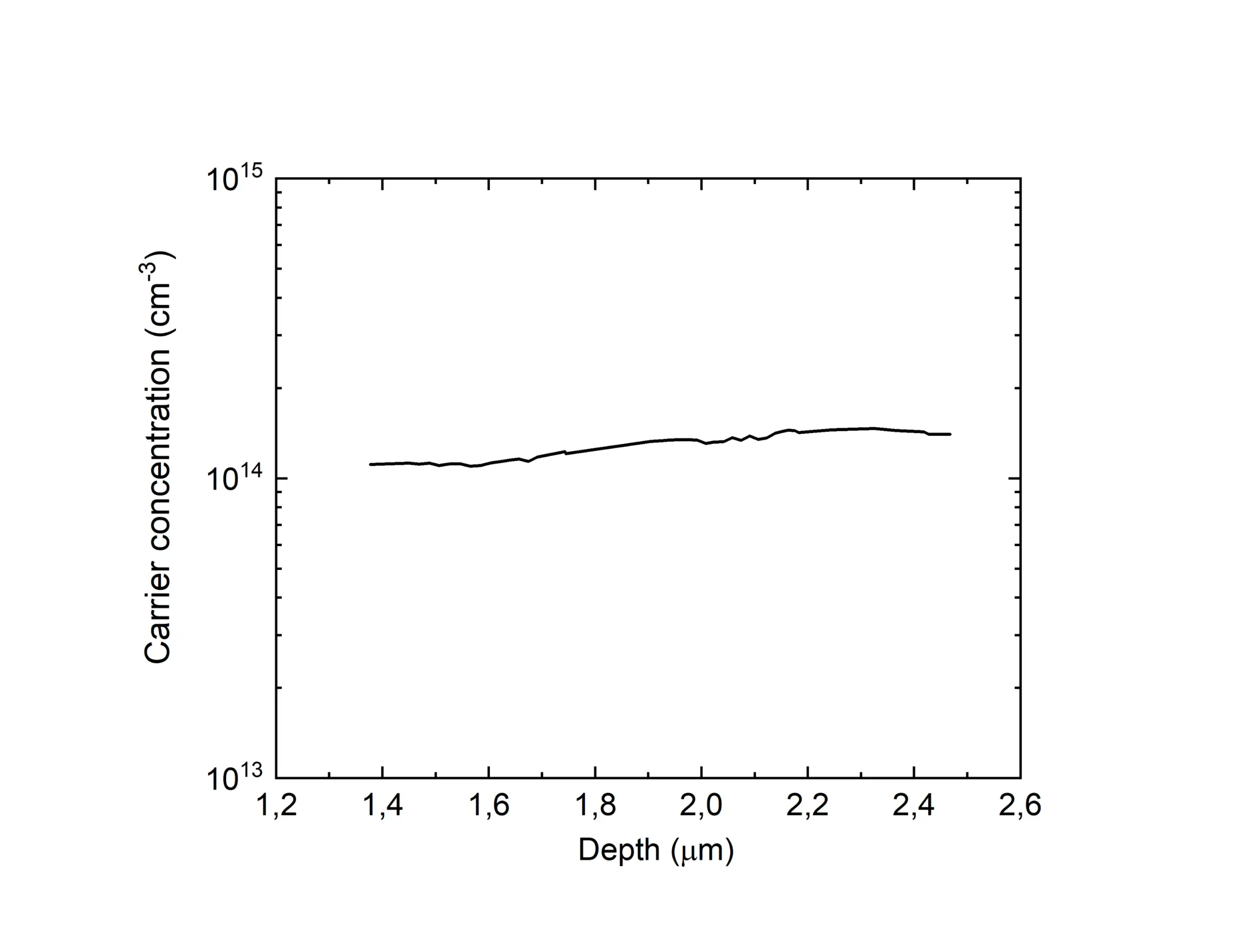
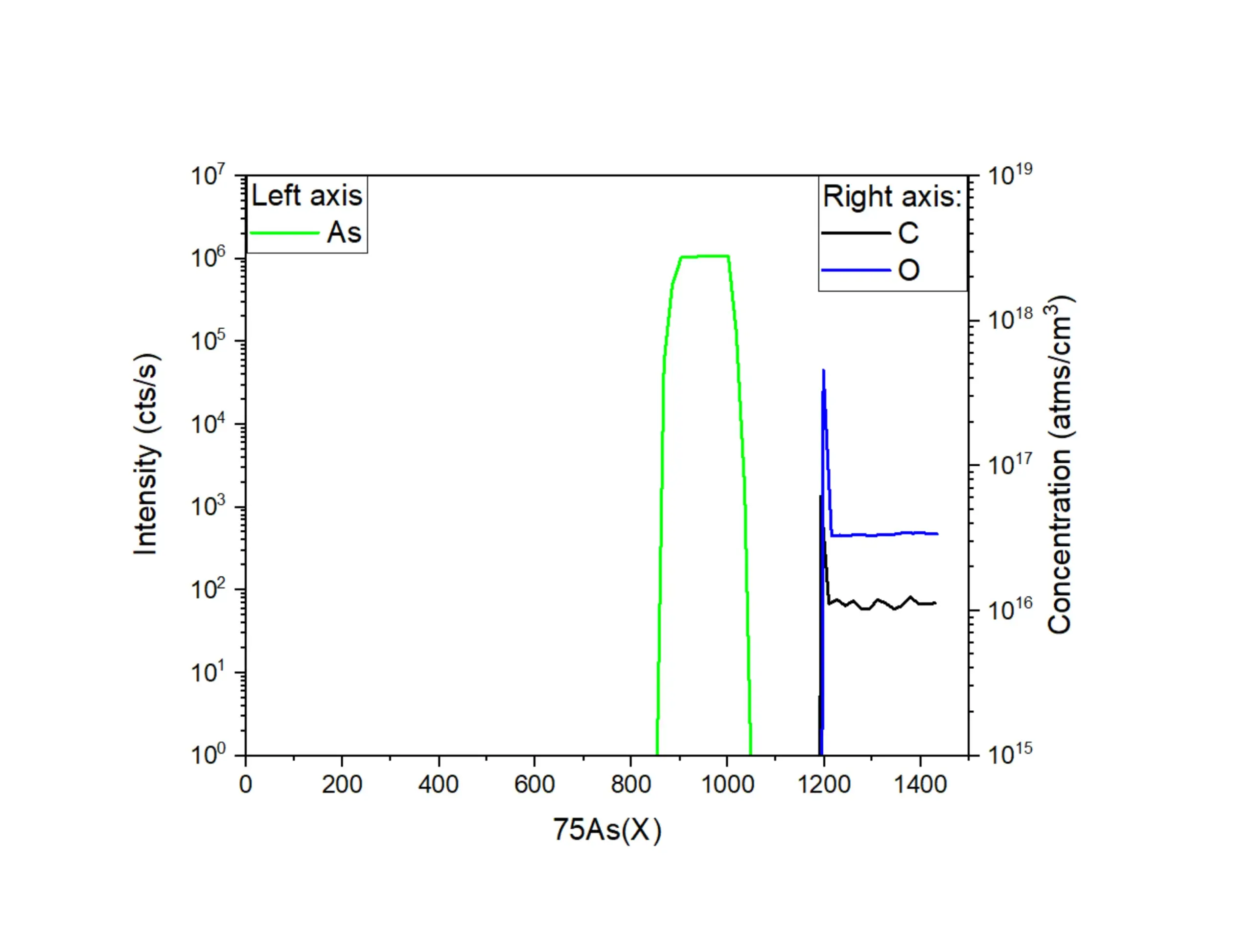
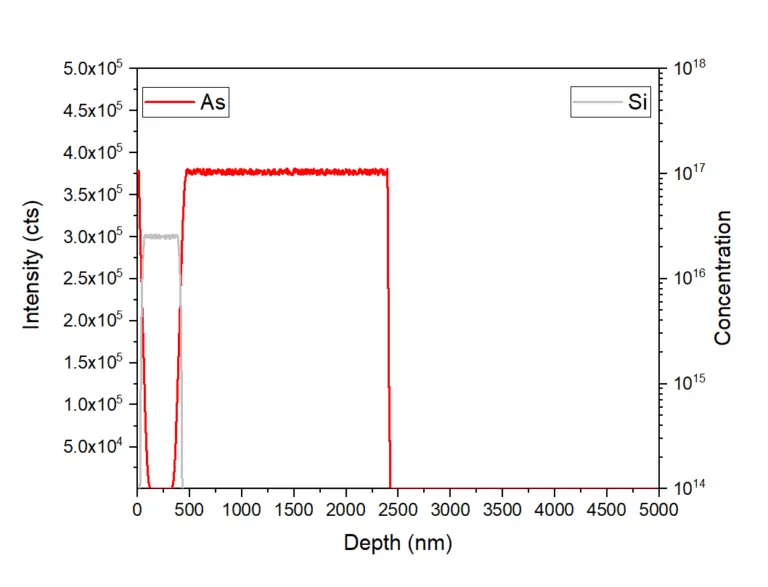
Layer thickness should also be optimized to a specific device parameters, according to the calculation at the epitaxial stack design phase. One of the best ways to control the thickness is through in-situ reflectance tools, such as LayTec. The chart presents the measured values that match the specification. For an after-growth verification a SEM measurement can be performed.
Finally, with a surface scan we assess the overall material quality. The most desired is the presence of atomic steps, which confirms the roughness at the level of single atomic layers. Another check is a calculation of the number of surface defects. In case of this measurement, the level of 2 defects per cm2 was achieved, which indicates almost defects-free material.
For Extended InGaAs the surface characterization should instead show a uniform cross-hatch as on the picture below: