Infrared detectors for space application
Several projects led by space agencies were built to expand our ability to observe many phenomena in space. Those projects are not limited only to the earth’s surface and earth’s atmosphere, which have a direct impact on human beings and the environment. Those are also extended farther away to observe nebulae, young stars, gas and dust in other galaxies, planets, and active galactic nuclei which need much effort to collect the data.
The techniques of infrared astronomy make it possible to investigate many astronomical phenomena. The character of the near-infrared spectrum is dust transparent, so it is able to see cool stars and other celestial objects. In far-infrared, the emission of the dust itself becomes observable. Relatively cold objects also emit most strongly in the infrared spectrum. Many gaseous molecules are readily visible in the infrared range, which have their unique ways of rotation and vibration. Thus, much infrared spectroscopy from space or near space with high altitude aircraft or remote objects, and also earth-based and earth-orbiting telescopes have been included in many space missions.
Different astronomical phenomena in the infrared range correspond with different methods of observation and require specific detection. VIGO Photonics is an expert in providing IR detectors and detector arrays from the SWIR to LWIR that support the space application. This paper describes some examples of the application of infrared detectors for space missions and the way to choose the best detectors provided by VIGO Photonics that suit your space exploration.
NIR | 0.7-1 µm | Star observing telescope | |
SWIR | 1-3 µm | Characterizing the spectral features of ground formations, Environmental mapping |
|
MWIR | 3-6 µm | Tracking the thermal emission | |
LWIR | 6-12 µm | Weather and climate monitoring from on-board satellites |
|
VLWIR | 12-30 µm | Weather and climate monitoring from on-board satellites |
|
FIR | 30-100 µm | Observing cold stellar objects in deep space |
Detector appropriate for space application
The detector appropriate for space application needs to have a wide spectral range with a small tolerance (+/- 1 μm) and the ability to enhance the optical signal. The detector also should pass several standard evaluations subjected to environmental screening, including thermal cycling, radiation, vacuum compatibility, burning, and vibration testing. Harsh environmental conditions in space by high-temperature fluctuations (-40oC to +85oC), shock, and vibration decrease the signal-to-noise ratio of the detectors. Thus, the detectors need to be cooled to give a stable measurement result at a high range of ambient temperature and have a low power operation. The detectors also need to have a short response time (< 6 s) for fast data acquisition and a long lifetime. To compromise with the small area of spacecraft and space satellites, the dimensions of the detectors also should be small and compact. Specifically for thermal sensing, the detector needs to be able to detect both cold and hot objects and be able to operate either sunlit or in shadow in a far distance.
HgCdTe | InAs / InAsSb / InGaAs | ||
Features | Can be operated in PC and PV mode | Mostly operated in PV mode | |
+ | - Best performance among all discovered materials- Tunable band-gap energy (2-30 μm)- High quantum efficiency in LWIR- Broad spectral range |
- Higher stability and uniformity across large area wafers
- Advance growth technology and manufacturability - Temperature stable up to 300 oC - Possibility of designing advanced structure as superlattices (SLs) |
|
- | - High cost of wafer growth and processing
- Less uniform across large area wafers |
- Cut-off wavelength at 7 μm
- Slightly worse performance than HgCdTe |
The main challenge for IR detectors for space appli- cation is to compete with the size of photographic film. Array technology made it possible to use larg- er numbers of detectors in a compact size in space focal plane arrays. The detectors can be arranged as linear sensor arrays or two-dimensional sensor arrays. VIGO Photonics provides detector arrays in the customized mode which consist of multiple spectral ranges that are appropriate for space application.
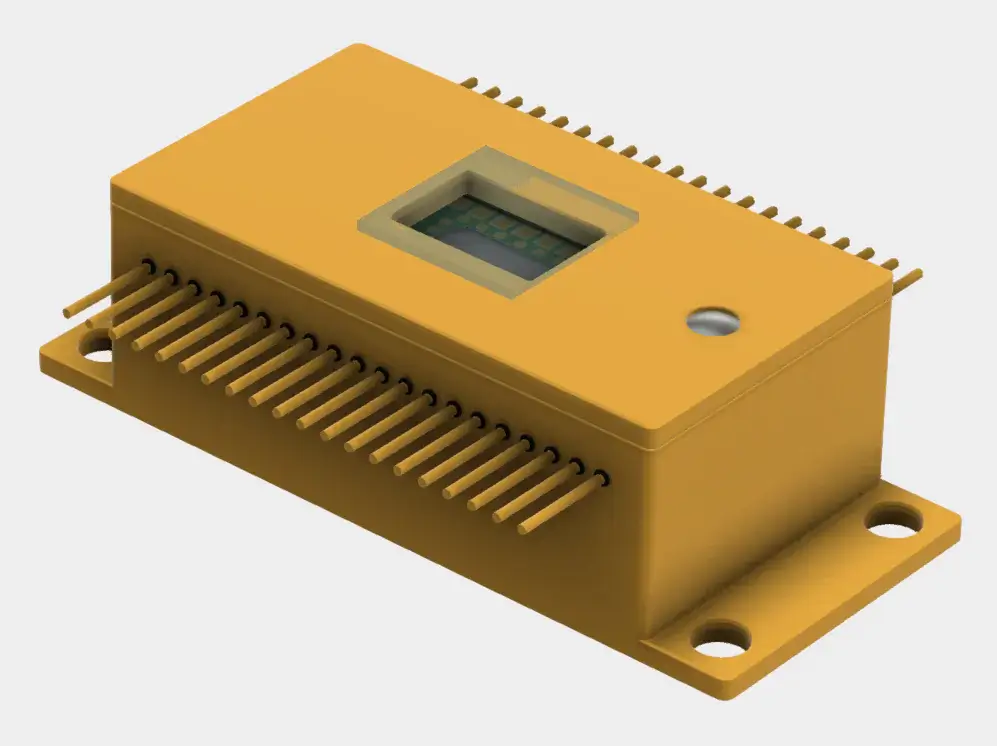
VIGO Detector Array Specification
Parameter | Value |
Detector material | HgCdTe or InAsSb |
Detector type | PV, PC |
Operating wavelength | MWIR (3.0 μm - 8.0 μm), LWIR (8.0 μm - 14.0 μm); Can be optimized upon request |
Pixel size | To be defined by the application; Minimum 25 μm sensor size |
Pixel pattern | To be defined by the application; Array or bi-array; Minimum 20 μm between pixels; Maximum 6 mm array length |
Enclosure/dimensions
|
TO8-16 pin, butterfly 40-pin |
TEC type | 2TE, 3TE |
Active elements temperature | 210 – 270 K |
Temperature sensor | Thermistor or diode (accuracy up to +/- 1 K) |
Cooler power | 1 - 4 W |
Time constant | 1 ns - 500 ns |
Window | Si/Al2O3/Ge with anti-reflection coating |
Ambient temperature | 0 to 70°C |
Storage temperature °C | -40 to 85°C |